Review of Petrochemical UV Filter Safety
- Dalia Mizikovsky
- Jan 2, 2020
- 9 min read
This report was published by Dalia Mizikovsky.
Funding for this report was provided by Advance ZincTek Limited, Brisbane.
Sunscreen is often used as the primary defense against sun damage and its harmful effects [1]. Despite the continuous increase in the use of sunscreen worldwide [2], most of the active ingredients lack the necessary safety data [3]. A Maximum Usage trial (MUsT) conducted by the US Food and Drug Administration (FDA) tested six active UV filters across four sunscreen formulations found that all petrochemical UV filters tested showed systematic absorption [5]. These findings regarding systemic absorption of petrochemical UV filters, raise unevaluated safety concerns on potential carcinogenic, reproductive and developmental effects [3,4].
These findings led the US FDA to re-evaluate the GRASE status of active ingredients in sunscreen [7]. GRASE is a measure used by the US FDA, which allows “drugs” to be sold over the counter without approved new drug applications, because they are generally recognized as safe and effective.
In a proposed rule, published on February 26, 2019 in the Federal Register – The Daily Journal of the United States Government [3] the FDA declared its review produced sufficient evidence that Zinc Oxide and Titanium Dioxide, two mineral UV filters used in sunscreen products met GRASE standards [6]. Trolamine Salicytate and Aminobenzoic acid (PABA) were determined by the FDA as not meeting GRASE standards [3], stating that the harm outweighed the benefit of their use [3].
In its proposed rule, the US FDA highlighted that for 12 petrochemical UV filters, there was insufficient safety information to conclusively determine the GRASE status (Table 1) [3]. In this report, we will review the results of the MUsT, in addition to other literature which has investigated the safety of petrochemical UV filters.
For non-prescription sunscreen products, the US FDA regulation for plasma concentration of a topically applied substance must be below 0.5 ng/ml in order to forego toxicology testing [6].
The threshold value of 0.5 ng/mL is based on the principle that that level would approximate the highest plasma level below which the carcinogenic risk of any unknown compound would be less than 1 in 100,000 after a single dose [6].
Table 1. Active sunscreen ingredients (UV filters) and their GRASE status as established in the proposed rule by the Food and Drug Administration on February 26, 2019 [3].

The MUsT found that every petrochemical UV filter tested exceeded this threshold after a single application (Table 2) [5]. Furthermore, the concentration of the petrochemical UV filters accumulated with subsequent application and remained above 0.5ng/ml in at half of the participants for at least 3 days after ceasing application [5]. Two of the UV filters, benzophenone-3 and homosalate were still measured to be above the 0.5 ng/ml threshold on day 21 of the study, 17 days after ceasing application [5]. Octocrylene had plasma concentrations that were 33-fold and 25-fold higher than the other UV filters tested at day 1 and day 4 of the MUsT respectively [5].
Previous studies have also identified the presence of petrochemical UV filters in other relevant biological samples, such as urine [8, 9], breast milk [10], the placenta [11], and cord blood [12]. It should be noted that physiological concentrations vary depending on the demographic sampled. Levels of UV filters in human samples are usually low [2, 5].
Table 2. Maximum Usage trial conducted by the Food and Drug Administration for six petrochemical UV filters. Sunscreen was applied once on day 1, and 4 times a day for the subsequent 3 days. Blood samples were collected multiple times per day for days 1 to 4, and then once on days 5 to 7, day 10, day 14 and day 21. [5]
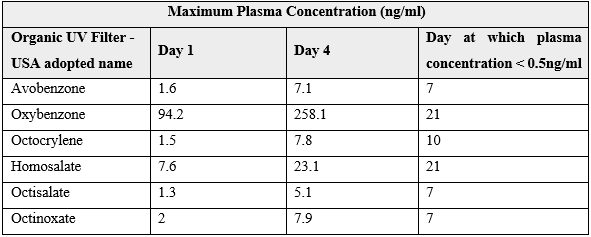
Dermal absorption is not the only relevant route of exposure to petrochemical UV filters [2]. UV filters also accumulate in bodies of water, benzophenone derivatives reaching concentrations of 300ng/ml in rivers, and at lower levels in sea and lake water due to the higher dilution factor [13, 14]. Furthermore, small amounts of petrochemical UV filters were found in tap and groundwater, suggesting ingestion as an additional route of exposure [13]. Swimming pools are another zone of major concern. They act as ‘sinks’ for petrochemical UV filters, and their chlorine byproducts, with concentrations reported as 2.85, 1.9, 1.78 and 0.95 g/L for EHMC, OC, 4-MBC and BP3 respectively [15].
Biological Activities of Petrochemical UV Filters
Endocrine disruption by petrochemical UV filters has been characterised in a range of in vitro and in vivo animal and human studies, finding that many petrochemical UV filters possessed some endocrine activity [16]. Benzophenones, camphor derivatives, and cinnamate derivatives, three of the major classes of petrochemical UV filters, have all been shown to possess estrogenic and androgenic disruptive effects, and to interact with the progesterone receptor in animal and in vitro studies [16-21]. Additionally, both benzophenones and cinnamate derivatives have been found to disturb the thyroid hormone receptor, and benzophenones the thyroid peroxidase enzyme [21-23]. These findings are reinforced by human studies, where small but significant changes in the relevant hormones were observed after topical application of sunscreen [24]. The low amplitude of the changes was partially attributed to robustness against endocrine changes in adults [24].
Minor endocrine changes are unlikely to be of detriment to adults [24]. However, children, whose developmental milestones are dependent on the correct endocrine signaling, are far more vulnerable to endocrine disruption [24, 25]. Exposure to petrochemical UV filters can begin as early as in utero with maternal use of sunscreen [26]. Maternal exposure to endocrine disrupting chemicals can affect the foetus via direct action if the foetus is exposed via placental transfer, or indirectly by the disruption of maternal hormones [26]. Placental transfer is likely, given the lipophilic nature of many petrochemical UV filters [27]. Indeed, petrochemical UV filters have been detected in the placenta, amniotic fluid, and cord blood [11, 29].
A human study from 2008, titled, Prenatal phenol and phthalate exposures and birth outcomes, [28] which investigated the effect of maternal urine concentration of benzophenone-3 on maternal hormones and birth outcomes found that the presence of benzophenone-3 was positively associated with birthweight in boys, and negatively associated with birthweight in girls [28]. A more recent study from 2018 [26] found a significant association between maternal urine and serum concentration of 4-hydroxybenzophenone (4-HBP), and decreased birth weight, head circumference and abdomen circumference [26]. Additionally, urine concentration of 4-HBP was positively correlated with maternal triiodothyronine (T3), thyroxine (T4) and insulin-like growth factor 1(IGF-1) [26]. 4-HBP is one of the known metabolites of benzophenones, in addition to a commercially used petrochemical UV filter, so a proportion of the serum and urine concentration may originate from other benzophenone filters [26].
There are several studies of the developmental toxicity of petrochemical UV filters using animal models which report significant effects from UV filter exposure [30-34].In one rat model, petrochemical UV filters 4-methylbenzylidene camphor and 3-benzylidene camphor was administered in food to the parent generation pre-mating, during pregnancy, and during lactation and then to the offspring until adulthood [10]. It was found that exposure to the petrochemical UV filters was associated with delayed puberty and enhanced prostate growth in males, and altered uterine gene expression in females [10]. Adult organ weights were altered in both sexes [10]. There are numerous other animal model studies of developmental toxicity of petrochemical UV filters which report significant effects from UV filter exposure [32-36].
In addition to exposure to petrochemical UV filters in utero, young children are also exposed through frequent topical application in a bid to prevent sun damage [35]. The absorption and resulting serum concentration of petrochemical UV filters, though not evaluated, may be higher in children than adults since children have a greater skin surface area to volume ratio [24]. Moreover, in the MUsT conducted by the FDA, it was proposed that the limiting factor for serum concentration of petrochemical UV factors was skin absorption, rather than elimination or breakdown of the compounds [5].
Endocrine disruptors can have a major impact on fetal growth, and pubertal development, in addition to being associated with conditions such as obesity [25]. However, the effects of petrochemical UV filters are not limited to endocrine action, several studies suggesting potential neurotoxic [2] and carcinogenic effects [36]. Moreover, recent evidence has shown that the breakdown products of petrochemical UV filters may be hazardous, opening up another avenue that requires investigation [37].
Further safety testing must be conducted, including thorough toxicology studies on all petrochemical UV filters, and their prominent breakdown products. Without this comprehensive information, a decision regarding their safety for further use cannot be made [3, 7].
ENDS
References
González S., Fernández-Lorente M, Gilaberte-Calzada Y. The latest on skin photoprotection. Clin Dermatol. 26, 614-26 (2008)
Ruszkiewicz, J. A. et al. Neurotoxic effect of active ingredients in sunscreen products, a contemporary review. Toxicol. reportst 4, 245-259 (2017).
Food and Drug Administration HHS. Sunscreen Drug Products for Over-the-Counter Human Use. 6204–6275 (2019).
Matta, M. K. et al. Effect of Sunscreen Application Under Maximal Use Conditions on Plasma Concentration of Sunscreen Active Ingredients. JAMA 321, 2082 (2019).
Matta, M. K. et al. Effect of Sunscreen Application on Plasma Concentration of Sunscreen Active Ingredients: A Randomized Clinical Trial. JAMA 323, 256–267 (2020).
Center for Drug Evaluation and Research. Nonprescription Sunscreen Drug Products — Safety and Effectiveness Data. Guidance for Industry. Silver Spring Maryland: Us Food & Drug Administration. (2016).
US Food & Drug Administration. FDA advances new proposed regulation to make sure that sunscreens are safe and effectiveitle. https://www.fda.gov/news-events/press-announcements/fda-advances-new-proposed-regulation-make-sure-sunscreens-are-safe-and-effective (2019).
Janjua, N., Kongshoj, B., Andersson, A.-M. & Wulf, H. Sunscreens in human plasma and urine after repeated whole-body topical application. J. Eur. Acad. Dermatology Venereol. 22, 456–461 (2008).
Rodriguez, J. & Maibach, H. I. Percutaneous penetration and pharmacodynamics: Wash-in and wash-off of sunscreen and insect repellent. J. Dermatolog. Treat. 27, 11–18 (2016).
Schlumpf, M. et al. Endocrine active UV filters: Developmental toxicity and exposure through breast milk. Chimia (Aarau). 62, 345-351 (2008)
Valle-Sistac, J. et al. Determination of parabens and benzophenone-type UV filters in human placenta: First description of the existence of benzyl paraben and benzophenone-4. Environ. Int. 88, 243–249 (2016).
Zhang, T. et al. Benzophenone-type UV filters in urine and blood from children, adults, and pregnant women in China: Partitioning between blood and urine as well as maternal and fetal cord blood. Sci. Total Environ. 461–462, 49–55 (2013).
Silvia Díaz-Cruz, M., Llorca, M., Barceló, D. & Barceló, D. Organic UV filters and their photodegradates, metabolites and disinfection by-products in the aquatic environment. TrAC Trends Anal. Chem. 27, 873–887 (2008).
Ramos, S., Homem, V., Alves, A. & Santos, L. Advances in analytical methods and occurrence of organic UV-filters in the environment — A review. Sci. Total Environ. 526, 278–311 (2015).
Sharifan, H., Klein, D. & Morse, A. N. UV filters interaction in the chlorinated swimming pool, a new challenge for urbanization, a need for community scale investigations. Environ. Res. 148, 273–276 (2016).
Wang, J. et al. Recent advances on endocrine disrupting effects of UV filters. Int. J. Environ. Res. Public Health 13, 1–11 (2016).
Schlumpf, M. et al. In vitro and in vivo estrogenicity of UV screens. Environ. Health Perspect. 109, 239–244 (2001).
Kunz, P. Y. & Fent, K. Multiple hormonal activities of UV filters and comparison of in vivo and in vitro estrogenic activity of ethyl-4-aminobenzoate in fish. Aquat. Toxicol. 79, 305–324 (2006).
Molina-Molina, J.-M. et al. Profiling of benzophenone derivatives using fish and human estrogen receptor-specific in vitro bioassays. Toxicol. Appl. Pharmacol. 232, 384–395 (2008).
Schreurs, R. H. M. M., Sonneveld, E., Jansen, J. H. J., Seinen, W. & van der Burg, B. Interaction of polycyclic musks and UV filters with the estrogen receptor (ER), androgen receptor (AR), and progesterone receptor (PR) in reporter gene bioassays. Toxicol. Sci. 83, 264–272 (2005).
Axelstad, M. et al. Effects of pre- and postnatal exposure to the UV-filter octyl methoxycinnamate (OMC) on the reproductive, auditory and neurological development of rat offspring. Toxicol. Appl. Pharmacol. 250, 278–290 (2011). y
Schmutzler, C. et al. The ultraviolet filter benzophenone 2 interferes with the thyroid hormone axis in rats and is a potent in vitro inhibitor of human recombinant thyroid peroxidase. Endocrinology 148, 2835–2844 (2007).
Schmutzler, C. et al. Endocrine disruptors and the thyroid gland--a combined in vitro and in vivo analysis of potential new biomarkers. Environ. Health Perspect. 115 Suppl, 77–83 (2007).
Janjua, N. R. et al. Systemic Absorption of the Sunscreens Benzophenone-3, Octyl-Methoxycinnamate, and 3-(4-Methyl-Benzylidene) Camphor After Whole-Body Topical Application and Reproductive Hormone Levels in Humans. J. Invest. Dermatol. 123, 57–61 (2004).
Meeker, J. D. Exposure to environmental endocrine disruptors and child development. Arch. Pediatr. Adolesc. Med. 166, E1–E7 (2012).
Krause, M. et al. Maternal exposure to UV filters: Associations with maternal thyroid hormones, IGF-I/IGFBP3 and birth outcomes. Endocr. Connect. 7, 334–346 (2018).
Hiller, J. et al. Systemic availability of lipophilic organic UV filters through dermal sunscreen exposure. Environ. Int. 132, 105068 (2019).
Wolff, M. S. et al. Prenatal phenol and phthalate exposures and birth outcomes. Environ. Health Perspect. 116, 1092–1097 (2008).
Krause, M. et al. Presence of benzophenones commonly used as UV filters and absorbers in paired maternal and fetal samples. Environ. Int. 110, 51–60 (2018).
Durrer, S., Maerkel, K., Schlumpf, M. & Lichtensteiger, W. Estrogen target gene regulation and coactivator expression in rat uterus after developmental exposure to the ultraviolet filter 4-methylbenzylidene camphor. Endocrinology 146, 2130–2139 (2005).
Durrer, S. et al. Estrogen sensitivity of target genes and expression of nuclear receptor co-regulators in rat prostate after pre- and postnatal exposure to the ultraviolet filter 4-methylbenzylidene camphor. Environ. Health Perspect. 115, 42–50 (2007).
Maerkel, K., Durrer, S., Henseler, M., Schlumpf, M. & Lichtensteiger, W. Sexually dimorphic gene regulation in brain as a target for endocrine disrupters: Developmental exposure of rats to 4-methylbenzylidene camphor. Toxicol. Appl. Pharmacol. 218, 152–165 (2007).
Maerkel, K., Lichtensteiger, W., Durrer, S., Conscience, M. & Schlumpf, M. Sex- and region-specific alterations of progesterone receptor mRNA levels and estrogen sensitivity in rat brain following developmental exposure to the estrogenic UV filter 4-methylbenzylidene camphor. Environ. Toxicol. Pharmacol. 19, 761–765 (2005).
Schlumpf, M. et al. Endocrine active and developmental toxicity of cosmetic UV filters – An update. Toxicology 205, 113-122 (2004).
Thoonen, K., Schneider, F., Candel, M., De Vries, H. & Van Osch, L. Childhood sun safety at different ages: Relations between parental sun protection behavior towards their child and children’s own sun protection behavior. BMC Public Health 19, 1–10 (2019).
Rhodes, M. C. et al. Carcinogenesis studies of benzophenone in rats and mice. Food Chem. Toxicol. 45, 843–851 (2007).
Wang, C. et al. Stability and removal of selected avobenzone’s chlorination products. Chemosphere 182, 238–244 (2017).
Comments